An overview of vaccine development & sub-types
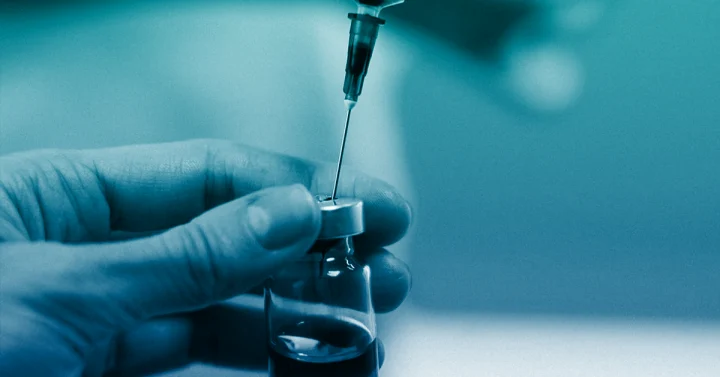
Vaccination can be regarded as one of biomedicines biggest triumphs. Since Edward Jenner pioneered the smallpox vaccine in 1798, subsequent morbidity and mortality rates have declined for a multitude of diseases, such as Diphtheria, Whooping Cough and Measles.1 However, despite the success of these global vaccination programs, continued research is still required to improve efficacy rates and safeguard us against emerging diseases, a hot topic with regards to the COVID-19 pandemic.2,3
Vaccines induce active immunity and immunological memory, enabling a rapid response upon later exposure from an infection.1,2 A Target Product Profile (TPP) is used to guide the vaccine development process early on. This document sets out clear parameters to help ensure the end-product is suited for its intended use and market. The key categories and considerations that may feed into a TPP include target population, route of administration, stability and storage, safety, and cost to name a few. 3
The basis of vaccine development historically focused on Louis Pasteur’s three I’s paradigm (isolate, inactivate and inject) although an improved understanding of immunology and microbiology has led to a more rational design and novel approach.1
Antigens provoking an immunogenic response can be engineered by a variety of techniques. Live attenuated vaccines contain whole, weakened viral or weakened bacterial cells, as used to fashion the Shingles vaccine. Inactivated vaccines contain whole or subunit parts of viruses or bacteria which cannot cause disease, such as polysaccharides or proteins. The Rabies vaccine is an example of a whole inactivated antigen. Further subtypes of subunit vaccines include toxoid, recombinant and conjugate and these were key in the generation of Diphtheria and Tetanus vaccines.1
COVID-19
The ongoing pandemic of COVID-19, caused by Severe Acute Respiratory Syndrome Coronavirus 2 (SARS-CoV-2), has led scientific institutions worldwide to direct their research towards developing a vaccine.3 Key elements of the TPP for a COVID-19 vaccine include suitability for adult healthcare workers, populations aged > 60 years as well as those with underlying health conditions including heart disease, diabetes and hypertension.
Several vaccine candidates are in the development process, ranging from live viruses to recombinant protein subunits. Eliciting an immune response against the Coronavirus S–protein to prevent its docking with the host ACE2 receptor is a strategy employed in the development of whole, subunit and nucleic acid-based vaccines.3
Another approach utilises the requirement of enveloped viruses to fuse their viral membrane with the host cell membrane for infection. Viral pre-fusion glycoproteins can elicit an immune response, although these are relatively unstable. Generating a recombinant viral protein to stably remain in pre-fusion form while inducing neutralising antibody production is in the development phase.4 Presenting itself as a global pandemic, the advancement of a successful vaccine is a priority alongside funding mechanisms to support manufacture and stockpiling.
Immuliver
The use of 3D in vitro models to study the lifecycle of viruses as well as routine assessment of vaccine attenuation is a popular alternative to animal testing.5,6 Research into the development of such robust and biologically relevant assays is ongoing particularly as live viruses including Hepatitis B and Yellow Fever analysis is at present heavily reliant upon animal models.7 A typical Yellow Fever assay for example, would use two sets of ten monkeys and this would be repeated up to four times a year, totalling approximately 80 animals.7 With respect to the aforementioned diseases, although several multicellular liver models are commercially available, they lack immunocompetency and further development is needed.5,6 A functional liver model requires defence against blood-borne infections, encompassing resident antigen presenting cells such as Kupffer cells, liver sinusoidal endothelial cells and hepatic stellate cells.
After winning an NC3Rs funded project, Newcells Biotech are developing an in vitro model that can be used for liver virus research, most specifically the routine assessment of Yellow Fever vaccine attenuation.8 Yellow Fever is a viral disease, spread via the bite of an infected female mosquito and causes symptoms ranging from fever and chills to liver damage and kidney failure. Currently, vaccine safety evaluation relies on animal models, for which the cynomolgus macaque is mostly used.9
Newcells Biotech aims to create a functional liver sinusoid model that represents the complex organ architecture required to successfully assess viscerotropism. The hiPSC-based model will be constructed from hepatocytes, stellate cells, endothelial cells and immune cells typically residing or invading liver cells during infection.8 An immunocompetent model will better recreate the in vivo microenvironment and physiological relevance of specific tissues. It will thus be better suited to research a multitude of liver diseases, ranging from viral infections, including Yellow Fever and Hepatitis B, to bacterial and parasitic infections such as granulomatous liver disease and plasmodium P.vivax respectively.10 This project highlights Newcells Biotech’s ongoing commitment to replace the use of animals in drug development with the generation of innovative in vitro assays. These human relevant models will additionally improve the predictive value of viruses and aid vaccine development, a science of which is notably important during the current COVID-19 pandemic.
References
- J Wallis, DP Shenton, RC Carlisle. Novel approaches for the design, delivery and administration of vaccine technologies. Vol 196, Clinical and Experimental Immunology. 2019.
- Han S. Clinical vaccine development. Vol 4, Clinical and Experimental Vaccine Research. 2015.
- W Chen, U Strych, PJ Hotez , ME Bottazzi. The SARS-CoV-2 Vaccine Pipeline: An Overview. Vol 3, Current Tropical Medicine Reports. 2020.
- YF Tu, CS Chien, AA Yarmishyn, YY Lin, YH Luo, YT Lin, WY Lai, DM Yang, SJ Chou, YP Yang, ML Wang, SH Chiou. A Review of SARS-CoV-2 and the Ongoing Clinical Trials. Vol 21, International Journal of Molecular Sciences. 2020.
- H Lee, S Chae, J Kim, W Han, J Kim, Y Choi, D Cho. Cell-printed 3D liver-on-a-chip possessing a liver microenvironment and biliary system. Vol 11, Biofabrication. 2019
- S Collins, G Yuen, T Tu, MA Budzinska, K Spring, K Bryant, NA Shackel. In Vitro Models of the Liver: Disease Modeling, Drug Discovery and Clinical Applications. In: Tirnitz-Parker JEE, editor. Hepatocellular Carcinoma [Internet]. Brisbane (AU): Codon Publications; 2019. Chapter 3. Available at: https://www.ncbi.nlm.nih.gov/books/NBK549191/ [Accessed May 2020].
- JC Moulin, J Silvano, V Barban, P Riou, C Allain. Yellow fever vaccine: Comparison of the neurovirulence of new 17D-204 StamarilTM seed lots and RK 168-73 strain. Vol 41, Biologicals. 2013
- Newcells Biotech Win Phase II of CRACK IT Challenge 29: ImmuLiver. December 2019 Available at: https://newcellsbiotech.co.uk/crack-it-challenge-29-immuliver/ [Accessed May 2020].
- T Monath, P. Vasconcelos. Yellow Fever. Vol 64, Journal of Clinical Virology. 2015.
- NC3Rs Innovative Platform, Challenge 29: ImmuLiver. Available at: https://crackit.org.uk/challenge-29-immuliver-0 [Accessed May 2020]
Share on social media:
Don't miss out on our latest innovations: follow us on Linkedin